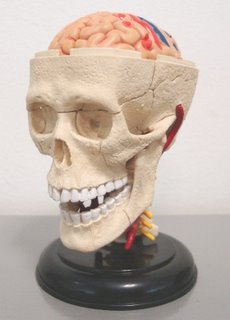
Around the mid-20th century he was concerned about the overemphasis on the reductionistic approach and the resulting fragmentation of science. In 1968 he published his book General System Theory where he wrote:
A consequence of the existence of general system properties is the appearance of structural similarities or isomorphisms in different fields. There are correspondences in the principles that govern the behaviour of entities that are, intrinsically, widely different. To take a simple example, an exponential law of growth applies to certain bacterial cells, to populations of bacteria, of animals or humans, and to the progress of scientific research measured by the number of publications in genetics or science in general.
System isomorphisms also appear in problems which are recalcitrant to quantitative analysis but are nevertheless of great intrinsic interest. There are, for example, isomorphies between biological systems and 'epiorganisms' like animal communities and human societies.
It seems therefore that a general system theory of systems would be a useful tool providing, on the one hand, models that can be used in, and transferred to, different fields, and safeguarding, on the other hand, from vague analogies which often have marred the progress in these fields.
Bertalanffy, together with some of his contemporaries, noted that regulation via feedback loops allow a system to maintain stability (today this field of study is called cybernetics).
He was also interested in the apparent contradiction between the 2nd law of thermodynamics and the increase in organizational complexity of living systems during embryo development and evolution. He proposed an idea that resolves this:
According to the second principle of thermodynamics, the general trend of events in physical nature is towards states of maximum disorder and levelling down of differences, with the so-called heat death of the universe as the final outlook, when all energy is degraded into evenly distributed heat of low temperature, and the world process comes to a stop.
In contrast, the living world shows, in embryonic development and in evolution, a transition towards higher order, heterogeneity, and organization.
But on the basis of the theory of open systems, the apparent contradiction between entropy and evolution disappears. In all irreversible processes, entropy must increase. Therefore, the change of entropy in closed systems is always positive; order is continually destroyed.
In open systems, however, we have not only production of entropy due to irreversible processes, but also import of entropy which may well be negative. This is the case in the living organism which imports complex molecules high in free energy. Thus, living systems, maintaining themselves in a steady state, can avoid the increase of entropy, and may even develop towards states of increased order and organization.
Bertalanffy's concept of an open system is usually illustrated like this:
Input refers to the stimuli and imported materials from the external environment, throughput refers to the processes within the system, and output refers to the resulting response or exported materials.
To emphasize the importance of feedback control, this version is also used:

Bertalanffy's open system model inspired biologist James Grier Miller to examine the applicability of systems theory to living systems.
Miller published Living Systems Theory in 1978, expounding his general theory about the existence of all living systems, their structure, interaction, behavior and development. He proposed that living systems must contain 20 critical subsystems which he later arranged into 8 nested hierarchical levels:
Miller noted that:
All nature is a continuum. The endless complexity of life is organized into patterns which repeat themselves—theme and variations—at each level of system. These similarities and differences are proper concerns for science. From the ceaseless streaming of protoplasm to the many-vectored activities of supranational systems, there are continuous flows through living systems as they maintain their highly organized steady states.
His observation of recursive patterns is likely to be inspired by the work of BenoƮt Mandebrot, the mathematician who founded the field of fractal geometry. Mandebrot observed that many objects in nature exhibited self-similarity and scale invariance - parts that are made up of smaller scale versions of the overall shape.
Interestingly, neither Bertalanffy nor Miller made any big impact on the field of biology itself, which in the wake of monumental discoveries of DNA and the central dogma, has remained firmly rooted in the reductionist paradigm.
General System Theory has become influential mainly in information science and cybernetics, whereas Living Systems Theory is more commonly read in sociology.
That is why as a neuroscience undergrad I had never heard of these guys and was completely unaware of systems theory, even though I regularly lamented with one of my fellow students about the lack of a systems interpretation of neuronal behaviour.
I first read Bertalanffy in 2005 when I picked up his General System Theory from the NUS library during the height of the popularity of the buzzword "Systems Biology". I wanted to know all this "systems" talk really meant, apart from expensive, shiny new high-throughput liquid handling robots.
I found his book to be quite repetitive but I was impressed by his open systems model, which I now call the "Bertalanffy Box". Excitedly, I wrote an article about it which was published in a student's magazine (GSS Journal 2005).
Here is an exerpt:
Actually, Systems Biology is not strictly a new idea. Some aspects of this perspective can be traced as far back as Plato! The modern synthesis of its fundamental concepts, however, first appeared in the General System Theory (GST) proposed by biologist Ludwig von Bertalanffy in the late 1940s. At that time, Bertalanffy was disturbed by what he saw as the overspecialization and fragmentation of science. He felt that the standard reductionistic approach to science was driving scientists to obsess over tiny details that may not advance the understanding of the big picture. His solution was to find some common patterns of organization in nature which could help unify the sciences and even the humanities together.
In a nutshell, the GST is a holistic theory that describes a complex system by examining the interactions between its components, rather than by analyzing the detailed structure of each component. Gesalt psychologists say that “the whole is greater than the sum of its parts,” an illustration which is also valid for the GST. In the context of biology, Bertalanffy described living organisms as “open systems” that interacts comprehensively with their environment. Next, he recognized that complex systems have emergent properties that cannot be predicted by knowing the properties of its components. In addition, he observed that such a system can also exert control over its components, such as in homeostasis, by using feedback loops.
Although I liked the simplicity of the Bertalanffy box, I found it to be incomplete and aesthetically displeasing. It is only focused on one particular system and has no scaleable aspects, thus it cannot take into account the inputs that might have arrived from another organization level, and ignores the outputs that will affect another organization level.
While recuperating from an illness in a hospital, I decided to improve the Bertalanffy box by integrating Bertalanffy's idea with Mandebrot's (I hadn't read Miller yet), and this is the result:
The "extended" Bertalanffy box (which I called a "leaky" open system at that time) features a system that is affected by both internal and external inputs and contributes outputs to both internal and external states. Thus, it is embedded in an organization level with constant interactions with lower, adjacent and higher levels.
Now I had a repeatable unit that is scaleable and conceptually self-similar.
To illustrate the self-similar aspect in a more visually striking manner, I designed this diagram about a year ago:
Here you can see a complex system opened to reveal the interactions in its intrinsic environment. It is made up of complex systems which are in turn made of smaller complex systems. With this simple repeating unit, you can model a system of any amount of complexity.
I hope I have clearly explained the "Fractal" aspect of FAMILIAR.
But what about the "Analog" part?
Stay tuned for my next post on heroes' journeys and multispecies alignments.
3 Comments:
So interesting, this was essentially my general paper question (well the feedback part) during my zoology honours almost two decades ago!
Hi Sivasothi, thanks for dropping by! Yes, I suppose Bertalanffy's ideas were already pretty old hat by the '80s. Feedback control is a crucial mechanism of maintaining system stability, but component level mechanisms such as self-organization may also play an important role.
Well, the question was set by a physiologist to whom feedback mechanisms are a second language, right next to ecologists.
We were to discuss feedback mechanisms at every level in three hours. I spent an hour thinking and then two generating spiel over 17 pages including 12 figures (specifics were needed).
This blog post made me realise that my extended introduction was simply reinventing the wheel, sheesh!
Nice idea for an exam anyway; they should bring it back.
Post a Comment